Research Summary and Impact
Transition Metal-free Graphite Intercalation Halide Cathodes
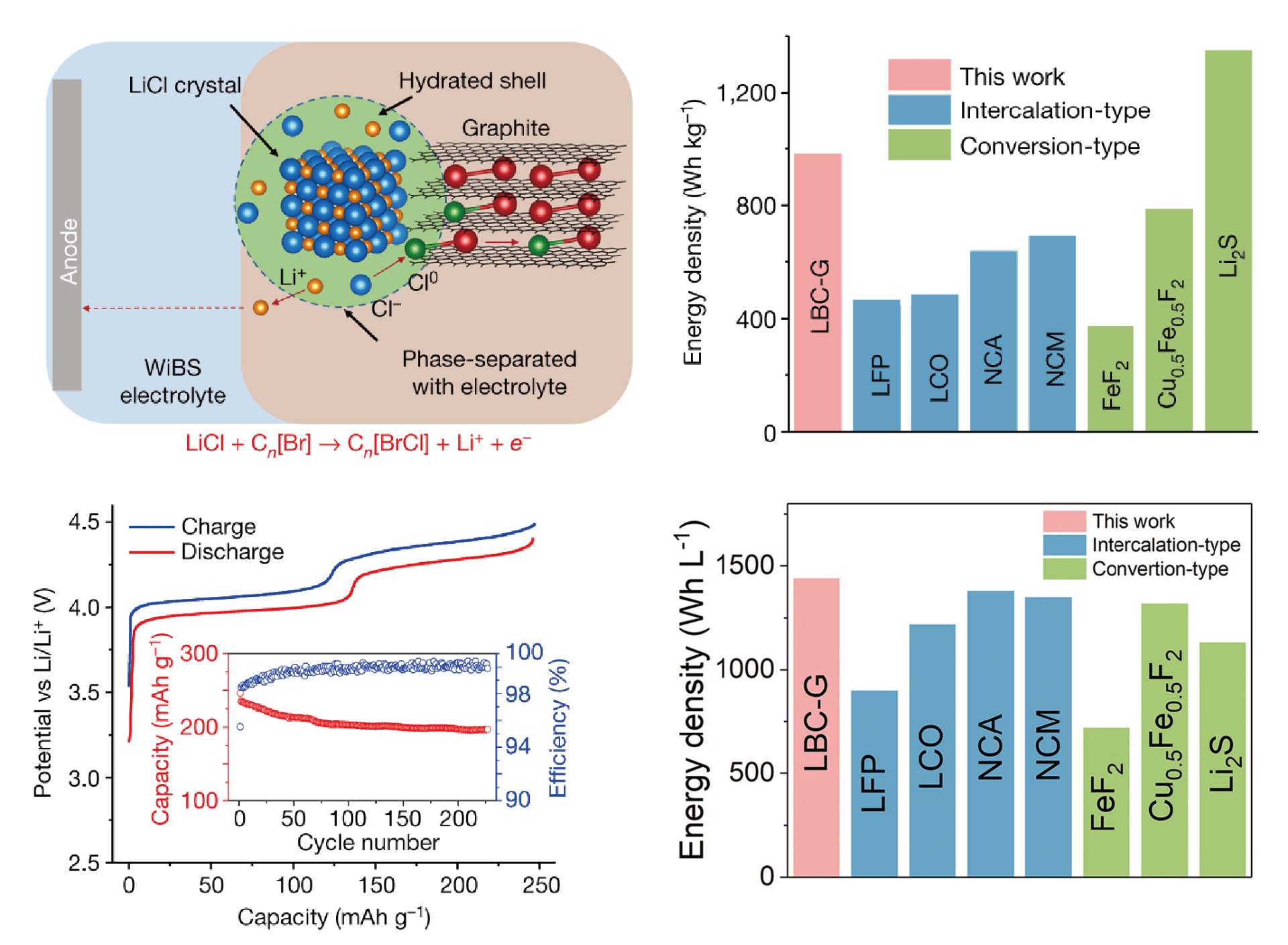
Conversion–intercalation mechanism and electrochemical performance of the LBC-G cathode in WiBS aqueous-gel electrolyte. The sequent oxidation and intercalation of Br- (about 4.0 V) and Cl- (about 4.2 V) into the graphitic in charge. The discharge is a reversal of the charge process.
Layered transition metal oxide LiNi1-x-yMnxCoyO2 cathodes are approaching their energy density limit, and the cost has been rapidly increasing due to the high price of Co and Ni. Dr. Wang and collaborators reported a transition metal free intercalation-conversion LiBr-LiCl-Graphite (LBC-G) cathodes that combine the high energy of conversion chemistries with the long cycle life of intercalation chemistries [1]. The working principle is that LiBr-LiCl absorb tiny water from water-in-salt electrolyte and becomes Li+ and Br–/Cl– on the LBC particle surface. During charge, Br– and Cl– intercalate in sequence into graphite cathode, and Li+ diffuse through water-in-salt electrolyte and then intercalate into graphite anode. The water-in-salt electrolyte is phase separated with LiBr-LiCl catholyte, preventing Br– and Cl– and their molecules diffuse to graphite anode side. The LiBr-LiCl-Graphite (LBC-G) cathode in water-in-salt electrolytes showed an energy density of 1000 Wh·kg-1 (capacity of 240 mAh·g-1 at 4.2 V) for 200 cycles. The gravimetric energy density of LBC-G is higher than current NMC cathodes, and volumetric energy density of LBC-G is higher than sulfur cathodes. Dr. Wang’s team has developed a non-aqueous electrolyte for LBC-G cathodes, now the LBC-G cathode can drop in the current Li-ion battery manufacturing.
Dr. Wang also made significant contribution on PAN-Sulfur, another transition-metal free cathodes, by increasing the sulfur loading in carbon to >60 wt % and sulfur utilization to >87% through dispersing sulfur in an oxygen-rich dense carbon host at a molecular level [2]. In an all-fluorinated organic lean electrolyte, the C/S cathode experiences a solid-state lithiation/delithiation reaction after the formation of solid electrolyte interphase in the first deep lithiation, completely avoiding the shuttle reaction. The chemically stabilized C/S composite retains a high reversible capacity of 541 mAh·g-1 (based on the total weight of the C/S composite) for 200 cycles under lean electrolyte conditions, corresponding to a high energy density of 974 Wh·kg-1 [2].
Representative publications:
-
C. Yang, J. Chen, X. Ji, T. P. Pollard, X. Lü, C. Sun, S. Hou, Q. Liu, C. Liu, T. Qing, Y. Wang, O. Borodin, Y. Ren, K. Xu, C. Wang, Aqueous Li-ion Battery Enabled by Halogen Conversion-Intercalation Chemistry in Graphite, Nature, 2019, 569, 245
-
C. Luo, E. Hu, K. J. Gaskell, X. Fan, T. Gao, C. Cui, S. Ghose, X-Q. Yang, C. Wang, A Chemically Stabilized Sulfur Cathode for Lean Electrolyte Lithium Sulfur Batteries. Proceedings of the National Academy of Sciences, 2020, 117, 14712-14
Multivalency Batteries for Renewable Energy Storage
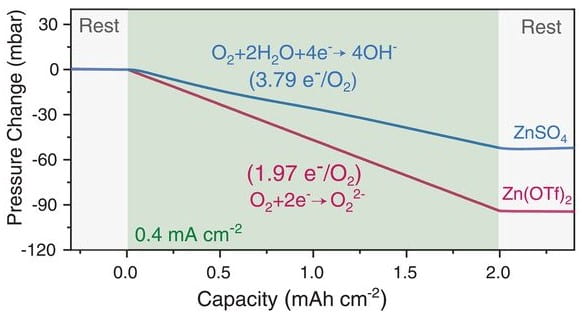
Pressure changes of Zn-O2 cells using Zn(OTf)2 and ZnSO4 electrolytes during a discharge process under neat O2 atmosphere
The electrochemical energy storage is being regularly deployed in the electricity grid to firm growing shares of renewables. Redox flow batteries (RFB) have been used for renewable energy storage because they have long operational lifetimes with low cost and can decouple the power and energy scaling. Most of the current RFB chemistries are based on expensive transition metal ions or synthetic organics. Dr. Wang’s group used a reversible Cl2/Cl– redox flow battery through electrolysis of aqueous NaCl electrolyte, the as-produced Cl2 is stored and extracted using mineral spirit. The membrane-free RFB design achieved an energy efficiency of >90.6% and energy density of 171 Wh·L-1, which are the highest among all flow battery systems reported [1]. With the inherently low cost of active materials (~5 $·kWh-1) and highly reversible redox reaction of Cl2/Cl–, the flow battery can meet the stringent price and reliability target for stationary energy storage.
In addition, low-cost multivalent batteries with earth abundant Zn, Mg, Al also potentially contend in renewable energy storage. Rechargeable aqueous zinc-air batteries suffer from Zn dendrite growth and the sluggish 4 electron (e–)/oxygen (O2) chemistry. For O2 cathodes, Dr. Wang’s group reported a zinc/O2 chemistry that proceeds through a 2e–/O2 process in nonalkaline aqueous electrolytes, which enables highly reversible redox reactions in zinc-air batteries. This ZnO2 chemistry was made possible by a water-poor and zinc ion (Zn2+) rich inner Helmholtz layer on the air cathode caused by the hydrophobic trifluoromethanesulfonate anions [2]. The nonalkaline zinc-air battery thus constructed not only tolerates stable operations in ambient air but also exhibits substantially better reversibility than its alkaline counterpart. To suppress Zn dendrite growth, Dr. Wang’s group designed a dilute and acidic aqueous electrolyte with an alkylammonium salt additive that enables the formation of a robust, Zn2+-conducting and waterproof SEI [3]. The presence of this SEI enables excellent performance: dendrite-free zinc plating/stripping at 99.9% coulombic efficiency in a Ti||Zn asymmetric cell for 1,000 cycles; and high energy densities (136 Wh·kg-1 in a Zn||VOPO4 full battery with 88.7% retention for >6,000 cycles, 325 Wh·kg-1 in a Zn||O2 full battery for >300 cycles and 218 Wh·kg-1 in a Zn||MnO2 full battery with 88.5% retention for 1,000 cycles) using limited zinc [3]. The low-cost aqueous Mg and Ca batteries can also meet the requirements for renewable energy storage [4].
Representative publications:
-
L. Chen, S. Hou, X. Fan, X. Ji, B. Wang, C. Cui, J. Chen, C. Yang, W. Wang, C. Wang, Nature Communications, revision submitted.
-
W. Sun, F. Wang, B. Zhang, M. Zhang, V. Kupers, X. Ji, C. Theile, P. Bieker, K. Xu, C. Wang, M. Winter, A rechargeable zinc-air battery based on zinc peroxide chemistry. Science, 2021, 371, 46-51.
-
L. Cao, D. Li, T. Pollard, T. Deng, B. Zhang, C. Yang, L. Chen, J. Vatamanu, E. Hu, M. J. Hourwitz, L. Ma, M. Ding, Q. Li, S. Hou, K. Gaskell, J. T. Fourkas, X-Q. Yang, K. Xu, O. Borodin, C. Wang, Fluorinated interphase enables reversible aqueous zinc battery chemistries, Nature Nanotechnology, 2021, 1730. Highlighted by Science, 28, 372, 890-891.
-
X. Tang, D. Zhou, B. Zhang, S. Wang, P. Li, H. Liu, X. Guo, P. Jaumaux, X. Gao, Y. Fu, C. Wang, G. Wang, A universal strategy towards high-energy aqueous multivalent-ion batteries, Nature Communications, 2021, 12, 2857.
Electrolyte Design for High Capacity Alloy and Lithium Anodes

Schematic of the cycled Si anode with an LiF-rich SEI coated on the surface
The EC-based carbonate electrolytes enable graphite to achieve a high Coulombic efficiency (CE) of >99.98% because the reduction of EC solvent (with minor contribution from anions) forms organic-inorganic solid electrolyte interphase (SEI) [1] that strongly bonds to micro-sized graphite (with low interface energy) and has sufficient plastic deformation capability to accommodate the small volume change (12%) of graphite during charge/discharge cycles. However, these strongly bonded organic-inorganic SEIs cannot withstand the large volume change of micro-sized alloy anodes or lithium metal (>300% for Si anodes and infinitive for lithium). Pre-lithiated nano-size Si at a high cost has to be used. Dr. Wang reported that the damage of SEI shell can be prevented by reducing the bond between SEI shell and alloy (or lithium) core, so that the inside alloy (or lithium) can freely shrink/expand during delithiation/lithiation. For Li anodes, the less bonding between SEI and Li also promotes Li growth along the Li/SEI interface rather than vertical to SEI because the high interface energy at SEI/Li largely increase energy penalty when Li ions penetrate into SEI. Among all SEI components, LiF SEI has lowest bonding to alloy and Li. Dr. Wang and his collaborators demonstrated that the LiF SEI can be formed in all-fluorinated [2] and 2.0 M LiPF6-mixTHF [3–4] electrolytes. The later electrolyte enables micro-sized alloys (Si, Al and Bi) [3] and Li [4] to achieve reordered high CE of >99.9% and 99.8% respectively. The CE of anion-derived LiF SEI for Li anodes also confirmed by PNNL groups using super-concentration and local-high-concentration electrolytes. Supported by DOE EERE, Dr. Wang’s group are extending the electrochemical stability window and reduce the thermos-stability of the electrolytes. By using fluorinated solvent and additives, Dr. Wang and his collaborators extended the stability window of non-flammable all-fluorinated electrolytes to 5.0 V [5], and enable Li/NCA batteries to operate from -90oC to +60oC [6]. This electrolyte design has been followed by many research groups and LiquaLith is commercializing the Si anodes. The electrolyte design principle for high capacity anodes are applicable to other batteries including K, Na, and Zn batteries.
Representative publications:
-
L. Wang, A. Menakath, F. Han, Y. Wang, P. Zavalij, K. Gaskell, O. Borodin, D. Luga, S. Brown, C. Wang, K. Xu, B. Eichhorn, Identifying the components of the solid–electrolyte interphase in Li-ion Batteries, Nature Chemistry, 2019, 11, 789.
-
X. Fan, L. Chen, O. Borodin, X. Ji, J. Chen, S. Hou, T. Deng, J. Zheng, C. Yang, S. Liou, K. Amine, K. Xu, C. Wang, Non-flammable Electrolyte Enables Li-Metal Batteries with Aggressive Cathode Chemistries, Nature Nanotechnology, 2018, 13, 715-722.
-
Chen, X. Fan, Q. Li, H. Yang, M.R. Khoshi, Y. Xu, S. Hwang, L. Chen, X. Ji, C. Yang, H. He, C. Wang, E. Garfunkel, D. Su, O. Borodin, C. Wang, Electrolyte Design for LiF-rich Solid-Electrolyte Interfaces to Enable High-performance Microsized Alloy Anodes for Batteries. Nature Energy, 2020, 5, 386–397.
-
Chen, Q. Li, T. P. Polland, X. Fan, O. Borodin, C. Wang, Electrolyte Design for Li Metal-free Li Batteries. Materials Today, 2020, 39, 118-126.
-
Chen, X. Fan, E. Hu, X. Ji, J. Chen, S. Hou, T. Deng, J. Li, D. Su, X. Yang, C. Wang, Achieving high-energy density through increasing the output voltage: a highly reversible 5.3 V battery, Chem, 2019, 5, 896.
-
Fan, X. Ji, L. Chen, J. Chen, T. Deng, F. Han, J. Yue, N. Piao, R. Wang, X. Zhou, X. Xiao, L. Chen, C. Wang, All-temperature batteries enabled by fluorinated electrolytes with non-polar solvents, Nature Energy, 2019, 4, 882.
Water-in-salt Electrolytes with High Electrochemical Stability Window
Dr. Wang and his collaborators have significantly extended the electrochemical stability window of aqueous batteries to 3.0 V by inventing the “water-in-salt” strategy to reduce the water activity and promote formation of solid electrolyte interphase [1]. The follow-up work revealed that the stability window could be further expanded to >3.3 V and a broad range of battery chemistries of LiMn2O4/sulfur [2], Zn-air [3], LiVPO4F/LiVPO4F [4] and LiVPO4F/G [5] have been demonstrated. Since in water-in-salt electrolyte, all the waters are bonded by Li-ion suppressing the water evaporation, and the oxygen has very low-solubility, an intrinsic safe open-cell with super-performance has been demonstrated using gel-water-in-salt electrolytes [6]. The gel-water-in-salt electrolyte LiMn2O4/Li4Ti5O12 open pouch cells are cut-able in the air [4], and the open cell technology has been selected by DOE ARPA-E to demonstrated in the Innovation Showcase on the Capitol Hill (Washington DC) in April, 2019 [7] and also in ARPA-E 10 years’ anniversary innovation summit at Denver on July 9th, 2019. In 2019 ARPA-E Innovation Showcase on the Capitol Hill, ARPA-E selected six ARPA-E teams to display their respective technologies for congressional staff members, industry stakeholders, and Department of Energy leaders. Recently, Dr. Wang’s team has reduced the salt concentration from 21m to 4.5m enabling LiMn2O4/Li4Ti5O12 pouch cells to achieve 1000 cycle life at P/N=1.14 and areal capacity of 1.5-2.5 mAh·cm-2 [8]. The scale-up of the 4.5m aqueous LiMn2O4/Li4Ti5O12 battery systems for special applications are currently underway by collaborating with Saft American and AquaLith with the funding from both Department of Defense and Department of Energy ARPA-E. This battery chemistry would remove the constraint of hermetical-seal on Li-ion batteries imposed by the moisture-sensitive non-aqueous electrolytes. After our Science publication, the water-in-salt electrolytes have been extensively investigated by the battery and electrochemistry community, with a number of leading groups in the world pursuing the fundamental and application of water-in-salt electrolyte for Li-ion, Na-ion, K-ion, Zn batteries.
Representative publications:
-
L. Suo, O. Borodin, T. Gao, M. Olguin, J. Ho, X. Fan, C. Luo, C. Wang, K. Xu, Water-in-Salt Electrolyte Enables High Voltage Aqueous Li-ion Chemistries, Science, 2015, 350, 938
-
C. Yang, L. Suo, O. Borodin, F. Wang, W. Sun, T. Gao, X. Fan, S. Hou, Z. Ma, K.l Amine, K. Xu, and C. Wang, Unique Aqueous Li-ion/Sulfur Chemistry with High Energy Density, Proceedings of the National Academy of Sciences, 2017,114, 6197–6202
-
F. Wang, O. Borodin, T. Gao, X. Fan, W. Sun, F. Han, A. Faraone, J. Dura, K. Xu and C. Wang, Highly Reversible Zinc-Metal Anode for Aqueous Batteries, Nature Materials, 2018, 17, 543-549
-
C. Yang, X. Ji, X. Fan, T. Gao, L. Suo, F. Wang, W. Sun, J. Chen, L. Chen, F. Han, L. Miao, K. Xu, K. Gerasopoulos and C. Wang, Flexible Aqueous Li-ion Battery with High Energy and Power Densities, Advanced Materials, 2017, 29, 1701972
-
C. Yang, J.Chen, T. Qing, X. Fan, W. Sun, A. v. Cresce, M. S. Ding, M.A. Schroeder, N. Eidson, C. Wang, K. Xu, 4.0 V Aqueous Li-ion Batteries, Joule. 2017, 1, 122–132
-
L. Chen, L. Cao, X. Ji, S. Hou, Q. Li, J. Chen, C. Yang, N. Edison, C. Wang, Enabling Safe Aqueous Lithium-ion Open Batteries by Suppressing the Oxygen Reduction Reaction. Nature Communications, 2020, 11,1-8
-
ARPA-E Innovation Showcase Recap.
-
J. Xu, X. Ji, J. Zhang, C. Yang, P. Wang, S. Liu, F. Chen, C. Wang, Aqueous Electrolyte Design for Super-Stable 2.5 V LiMn2O4||Li4Ti5O12 Pouch Cells, Nature Energy, revision submitted.
Solid State Electrolytes: Li Dendrite Formation and Suppression
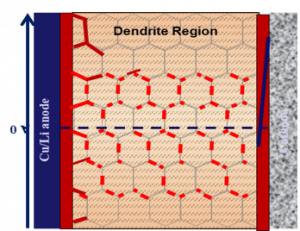
Li dendrite deposition inside solid state electrolyte
Solid electrolytes (SEs) are widely considered as an ‘enabler’ of lithium anodes for high-energy batteries. However, recent reports demonstrate that the Li dendrite formation in Li7La3Zr2O12 (LLZO) and Li2S-P2S5 (LPS) is actually much easier than that in liquid electrolytes of lithium batteries, by mechanisms that remain elusive. Dr. Wang identified the lithiophilic SEIs formed in LLZO and LPS solid state electrolytes as the main reason that promotes Li dendrite [1, 2], as opposed to the lithophobic LiF-rich SEI formed in liquid fluorinated electrolytes. In addition, the solid state electrolytes (LLZO and LPS) also have a much higher electronic conductivity (10-9 S·cm-1) than a liquid organic electrolyte (insulator), which can potentially reduce the potential of solid-state electrolyte to <0.0 V at a high Li plating current, resulting in Li deposition inside SSEs [3, 4]. Based on the Li dendrite formation mechanism, Dr. Wang introduced a thin lithiophobic Li3N-LiF interlayer between LPS and Li, which increased the critical current to 6.0 mA·cm-2 at a high capacity of 6.0 mAh·cm-2 due to the effective suppression of the Li dendrite growth [4]. The lithiophobic interlayer, which is either ionic or mixed electronic/ionic conductor, can prevent the Li deposition on the solid-state electrolyte surface. Therefore, the potential of the solid-state electrolyte will not drop to Li deposition potentials inside electrolyte. In addition, the lithiophobic interlayer can also prevent Li penetration into solid state electrolytes. The proposed Li dendrite suppression mechanism has also been validated by Samsung [Nature Energy, 5, 299–308(2020)] with a mixed conductive lithionphobic carbon-silver interlayer between Li and LPSCl, where Li did not deposit on the surface LPSCl but on the bottom of C-Ag layer. Many leading research groups in the world are using the proposed mechanism to suppress the Li dendrite growth in solid-state electrolytes.
Due to the poor contact between solid current collector and solid electrolyte, the electrochemical stability of the solid-state electrolytes measured using traditional blocking electrode is wider than the actual value. Dr. Wang enhanced the accuracy using a 3D current collector by adding electronic conductor into solid-electrolyte [5].
Representative publications:
-
Ji, S. Hou, P. Wang, X. He, N. Piao, X. Fan, C. Wang, Solid-State Electrolyte Design for Lithium Dendrite Suppression, Advanced Materials, 2020, 32, 202002741
-
Fan, X. Ji, F. Han, J. Yue, J. Chen, L. Chen, T. Deng, J. Jiang, C. Wang, Fluorinated solid electrolyte interphase enables highly reversible solid-state Li metal battery, Science Advances, 2018, 4, eaau9245
-
Liu, R. Garcia-Mendez, A. R. Lupin, Y. Cheng, Z. D. Hood, F. Han, A. Sharafi, J. C. Idrobo, N. J. Dudney, C. Wang, C. Ma, J. Sakamoto, M. Chi, Local electronic structure variation resulting in Li ‘filament’ formation within solid electrolytes, Nature Materials, 2021, https://doi.org,10.1038/s41563-021-01019-x
-
Han, A. Westover, J. Yue, X. Fan, F. Wang, M. Chi, D. Leonard, N. Dudney, H. Wang, C. Wang, High Electronic conductivity as the origin of lithium dendrite formation within solid electrolytes, Nature Energy, 2019, 4, 187-196
-
Han, T. Gao, Y. Zhu, K. J. Gaskell, C. Wang. A Battery Made from a Single Material. Advanced Materials, 2015, 27, 3473
Research Center Leadership and Entrepreneurship
Founded a National Center for Research in Extreme Batteries (CREB)
Dr. Wang is a co-founder and serving as the UMD Director and steering committee member for CREB. The consortium of CREB includes 5 government Labs (ARL, National Institute of Standard and Technology, Naval Research Lab, NASA, Argonne National Lab), 5 Universities (UMD, UMBC, JHU, UVA, Drexel), and 5 industries (Saft, DuPont, NoHMs Technologies, NY BEST Technology, Lockheed-Martin). The purpose of the center is to foster collaboration on both fundamental and applied battery materials research aiming at defense, space and medical applications. CREB received congressional funding of $10 M in 2020, and $10 M in 2021.
Entrepreneurship
In March 2020, Dr. Chunsheng Wang founded WISE Batteries Inc, which is a spinout company from research conducted by Dr. Wang at the University of Maryland. AquaLith Advanced Materials, Inc licensed Dr. Wang’s battery Technology to commercialize next generation of high energy batteries.